an email newsletter released every month highlighting the latest articles, events, technical inquiries, and voices from the community
6G and Its Advancements Over 5G Networks

Posted on August 3, 2023 | Completed on February 15, 2023 | By: Olutoye Sekiteri
What major advancements are proposed by 6G, and when is it projected to deploy?
The Cybersecurity and Information Systems Information Analysis Center (CSIAC) was tasked with researching and recognizing improvements to 6G networks compared to 5G. The inquirer also wanted to know when 6G networks are expected to be deployed. CSIAC identified the differences in features and functionality between 6G and 5G networks, projected 6G network deployment timeframes, and potential future use cases for 6G technologies.
1.0 TI Request
1.1 Inquiry
What are the planned major advancements proposed by 6G, and when is this new cellular evolution projected to deploy?
1.2 Description
The Cybersecurity and Information Systems Information Analysis Center was asked to provide information on the technological advancements of 6G networks compared to 5G networks and when to expect deployment of 6G networks for the United States and abroad. The inquirer noticed that early planning was beginning for 6G networks and wanted to know more about what 6G intends to provide.
2.0 TI Response
2.1 What Is 6G?
6G is the sixth generation of wide-area wireless technologies. The 6G wireless standard is meant to improve many of the features of its predecessor, 5G, including operating on a higher-band radio spectrum, efficient energy consumption, greater scalability, less latency, more speed, the ability to access different connection types dynamically, the addition of artificial intelligence (AI) and machine-learning (ML) capabilities by design, and more. 6G is the successor to 5G cellular technologies, but it is not necessarily designed to replace 5G completely. 6G and 5G networks should be considered different versions or modes of wireless connectivity, meaning they will work in tandem to fulfill separate use cases. Transitioning all our current devices to a 6G infrastructure does not seem to be practical for the initial rollout of the technology. 6G will first be focused on the business, military, and industrial sectors and will only be useful for some consumer products and services that can take advantage of the technology, such as immersive entertainment.
Major research and development of 6G technologies started in 2020. To launch 6G networks commercially, advanced mobile communication technologies, edge computing, AI, ML, a more connected Internet of Things (IoT), energy harvesting/transfer capabilities, and more will have to be developed and implemented into the new wireless standard. In addition, current spectral bandwidth capabilities will also have to be expanded. China has already launched a 6G test satellite equipped with a terahertz system [1]. However, it is predicted that 6G technology will not launch commercially until 2030 [2].
6G technology is expected to achieve the following requirements in the future [3]:
-
- Very low latency communication (10–100 µs)
- A peak data rate of at least 1 TB/s
- Support high mobility (≥1000 km/h) to support aircraft and air systems
- Huge device capacity (107 device/km2)
- Reduce energy consumption to introduce green communication (100× energy reduction compared to 5G)
- Data rate reaches 10 GB/s (10× higher than 5G)
- 99.99999% availability
- Spectrum efficiency (5–10× more efficient than 5G)
- High three-dimensional (3-D) coverage extension, including terrestrial, aerial, space, and sea domain
2.2 Advancements of 6G Compared to 5G networks
2.2.1 Spectrum Usage
5G and 6G networks both operate using wireless signals on the higher end of the spectrum to obtain data transmission rates that are higher than 4G and previous generations (Figure 1). 5G is reserved for low- and high-band frequencies that are sub 6 GHz and above 24.25 GHz, respectively. 6G networks will operate within the ranges of 95 GHz to 3 THz in frequency. The use of terahertz frequency bands allows 6G networks to obtain data transfer rates higher than what is possible with 5G networks. Due to the difference in the spectrum frequency range used, both 5G and 6G networks will be utilized for different use cases in a variety of industrial sectors to improve overall efficiency of operations.
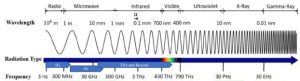
Figure 1. The Electromagnetic Spectrum (Source: https://www.osti.gov/servlets/purl/1838194).
2.2.2 Speed and Latency
Considering the performance of these networks, 6G will be able to provide much higher performance compared to 5G networks. 6G networks will be able to achieve this with the use of terahertz frequency bands. It is still a bit too early to accurately predict the future transmission rates of 6G networks. However, early estimates claim 6G networks have the potential to give users speeds of 1 TB/s, or 8,000 GB/s, according to Dr. Mahyar Shirvanimoghaddam, an expert in wireless communication at the University of Sydney [4]. When we talk about 5G-vs.-6G network speeds, 6G networks are expected to be 100× faster than 5G, with enhanced reliability and wider network coverage.
Latency is the time it takes a packet of information to transmit over a frequency. 4G networks operate with a latency of about 50 ms. 5G made improvements upon 4G’s transfer speeds having latency 10× lower than 4G at 5 ms. 6G internet is expected to improve upon 5G even more and provide transmission speeds in a range of anywhere from 1 ms to as low as 1 µs. This means 6G has the potential to reduce latency at least 5× lower, compared to 5G.
The use of submillimeter waves (wavelengths less than 1 mm), terahertz frequency bands, and reduced packet transmission rates is expected to advance the development of wireless sensing technology, real-time computing, and network reliability and open the door for huge data transmission capabilities.
2.2.3 Expansion of IoT Capabilities
As the number of connected devices continues to grow (estimated to reach 55.7 billion by 2025), improvements in data transfer speeds manifest and the implementation of 5G-based solutions continue to increase, further developing the IoT becomes a real possibility. IoT, the deployment and interconnectivity of devices far beyond personal cellular devices, includes, but is not limited to, household appliances, medical devices, cars, power and transportation infrastructure, and logistics management devices (Figure 2). This influx of devices is projected to generate 73.1 ZB of data (the equivalent of 1.9 million iPhones), necessitating new technological advances in data transfer and storage [2]. This was previously impossible with older networks like 4G long-term evolution due to improper frequency planning and management. The frequencies used by older networks were too congested, constrained, and narrow for the stable data transmission rates required by smart devices to function properly. 5G networks have been able to fill the gap to facilitate the use and deployment of IoT devices. 5G’s support of IoT has made it a practical everyday reality for users. As research and development of 6G continues, 6G networks anticipate improving current capabilities with 5G networks and connecting 10× more IoT devices per square kilometer.
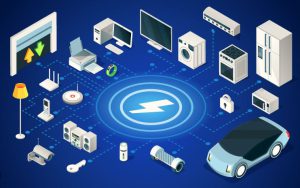
Figure 2. IoT (Source: https://standby.lbl.gov/).
According to a new study by IHS Markit, a worldwide leader in critical information, analytics, and solutions, the number of linked global IoT devices will grow at a rate of 12% per year, from about 27 billion in 2017 to 125 billion in 2030 [5].
In this perspective, 6G will be a significant enabler for future IoT systems and applications, as it will provide full-dimensional wireless coverage and integrate all functionality, including sensing, transmission, computation, cognition, and fully automated control [6].
2.2.4 AI and ML
5G Advanced, predicted to be deployed by 2025, is a planned set of technological and network upgrades that will expand on the existing capabilities of 5G. One key component of 5G Advanced is the application of AI and ML solutions to introduce more intelligent network management capabilities and solve multidimensional optimization issues that come with real-time/nonreal-time network operation. This will help manage the expected increase in connected devices, enabling higher download speeds and preserving low latency [7]. The partial implementation of AI/ML capabilities into 5G will push wireless networks forward, but 6G’s full support of AI/ML for automatization by design will be revolutionary.
6G networks are expected to support centralized high-performance computing (HPC) and bring that form of computing to the end terminals. The use of HPC will provide 6G networks with the ability to not only be intelligent but self-sufficient. This allows for the implementation of distributed learning models within the network, which promotes increasing efficiency, reducing processing time within communications, solving complex problems efficiently, using a smart self-sustaining wireless network, and making real-time network decisions through prediction.
Edge computing, moving servers and data processing closer to connected devices, could help with data storage while still maintaining 6G speeds and low latency (Figure 3). Due to the large migration of computing infrastructure to the cloud, mobility-enhanced edge computing (MEEC) will be an important part of 6G technologies. Developing MEEC infrastructure with AI capabilities opens the door for effective computing of big data analytics and devices/processors at the edge. 6G’s application of AI-based technology can be characterized under the following types of key performance indicator-associated services: ubiquitous mobile ultra-broadband (uMUB), ultra-high speed with low latency communications (uHLSLLC), massive machine-type communication (mMTC), and ultra-high data density (uHDD) services. Having a strong edge infrastructure connected to 6G networks could facilitate the implementation of AI/ML on a massive scale, leading to smarter devices that can aid decision makers with its dynamic capabilities.
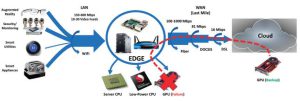
Figure 3. Using Edge Computing to Process Video and Compensate for Edge Graphics Processing Unit Failure (Source: https://par.nsf.gov/servlets/purl/10197259).
On a microscale, these applications can be used for autonomous vehicles to improve traffic flow and allow the modernization of public transportation with autonomous taxi and bus services in cities. On a macroscale, manufacturing, logistics, and analytical industries will be able to improve efficiency as AI and ML find trends and paths unseen by humans, speeding up supply chains and product production. For local, state, and federal governments, services can be metered and controlled to match demand more precisely at any given moment, increasing efficiency and improving availability, and slowing the effects of global warming. Additionally, these algorithms could aid in predictive modeling for services (e.g., Department of Motor Vehicles wait times, trash collection, and bus usage) and weather events (e.g., tornados, floods, and fires) [2].
2.2.5 Energy Harvesting and Backscatter Communications
Most devices applying 6G will dissipate much more energy than previous generations because of the enormous capacity that will work in higher frequency bands. It is becoming more desirable to empower battery-limited devices by using energy harvesting and wireless energy transfer as technology advances toward 6G [3]. Some examples of battery-limited devices are smart-farming sensors, health-monitoring implants, sensors for critical infrastructure like power plants, and hardware in unmanned aerial systems (UASs). Given the scale expected in 6G networks, it is necessary to design systems where both the circuitry and the communication stack are developed with energy awareness. One option is using energy-harvesting circuits to allow these battery-limited devices to be self-powered, which could be critical. For example, energy harvesting can facilitate off-grid operations, long-lasting IoT devices and sensors, or long stand-by intervals for devices and equipment rarely used [8]. Being able to harvest energy to devices through various methods such as dedicated radio frequency (RF) harvesting sources, interference-aware harvesting, and ambient-sunlight harvesting will help improve the operational longevity of many smart systems and reduce the amount of maintenance for systems that implement 6G.
Ambient backscatter wireless communications allow interaction between two batteryless devices by using existing RF signals such as ambient television and cellular transmissions. A viable data rate can be obtained at shorter communication ranges. Transmissions of small monitoring signals can also be transferred with little-to-no-power consumption. Backscatter communications gives battery-limited or batteryless devices the ability to be self-sufficient and long lasting in off-grid and remote environments.
2.2.6 Holographic Beamforming
Beamforming is a procedure based on the signal processing in which a radio signal can be transmitted in precise directions using an array of steered antennas through the emphasis on the minimized angular range. Beamforming has been implemented with 5G networks to provide higher network efficiency, better coverage/throughput, higher signal-to-inference noise ratio, user tracking, interference prevention, and rejection. Holographic beamforming (HBF) is a new method based on the usage of a hologram to achieve beam steering through the antenna. In this method, the RF signals emerging from the radio travel to the back of the antenna, scatter across its front, and later adjust according to the beam shape and direction. Based on using software-defined antennas (SDAs), this method is quite different from multiple input multiple output (MIMO) systems because SDAs are smaller, lighter, cheaper, and low-power consuming compared to the traditional arrays or MIMO systems [6]. Using SDAs in the HBF procedure will lead to highly flexible and efficient transmission and reception of signal in the 6G networks.
For the 6G networks, using the HBF approach in multiantenna communication devices is advantageous for transmitting and receiving signals in a highly efficient and flexible manner. This indicates important roles of HBF positioning in the wireless power transfer, physical layer security, and augmented network coverage related scenarios [6].
2.2.7 Blockchain
Blockchain is an essential technology to manage massive data in future communication systems. Blockchains are just one form of distributed ledger technology. A distributed ledger is a database that is distributed across numerous nodes or computing devices around the world. Each node replicates and saves an identical copy of the ledger. Peer-to-peer networks manage the blockchain, which can exist without being controlled by a centralized authority or a server. The data on a blockchain are gathered and structured in blocks, which are connected and secured using cryptography. The blockchain is a perfect complement to the massive IoT, with improved security, privacy, interoperability, reliability, and scalability. Therefore, blockchain technology will provide several facilities, such as interoperability across devices, traceability of massive data, autonomic interactions of different IoT systems, and reliability for the massive connectivity of 6G communication systems to reach the goal of uHSLLC service.
Blockchain builds trust between networked applications and voids the necessity of trusted intermediaries. The integral features of blockchain, such as decentralized tamper-resistance and secrecy, make it ideal for numerous applications in 6G communication. It creates a secure and verifiable approach for spectrum management by establishing transparency, verified transactions, and prevention of unauthorized access. Blockchain combines a distributed network structure, consensus mechanism, and advanced cryptography to represent promising features unavailable in existing structures. The distributed nature eliminates the single point of failure problem and enhances security. The main challenge of blockchain networking in 5G is the throughputs (10–1000 transactions per second). Another challenge is the demand for local and international standardization and regulation of the massive adoption of blockchain in 5G. Still, 5G considers the issue of smooth interoperability between different blockchain platforms. These limitations can be mitigated in 6G by using consensus algorithms, applying novel blockchain architecture and sharing techniques, and increasing the block size of the network [9].
2.3 Potential 6G Use Cases for the Future
2.3.1 Autonomous Vehicles
Public Transportation
As the U.S. government plans to end the purchase and development of gas-powered vehicles by 2035, it is safe to say that the future of cars will include an increased amount of electric and autonomous vehicles [10]. There are six levels of autonomous cars, including levels 0, 1, 2, 3, 4, and 5. The difference in levels of cars is their varying automation levels, i.e., level zero has no automation, whereas level 5 represents full automation. Moreover, these cars can handle lane changing and tackle collision avoidance [6]. They will consist of buses, taxis, semi-trailers, and commercial and consumer vehicles. As time goes on, this new generation of vehicles will continue to make up a larger percentage of transportation on the road (Figure 4).
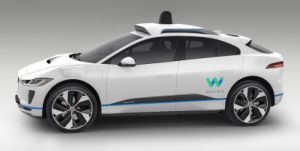
Figure 4. Waymo Autonomous Jaguar I-Pace Electric Vehicle (Source: https://www.energy.gov/eere/vehicles/articles/fueling-infrastructure-future-shared-and-shared-automated-vehicles).
The evolution toward fully autonomous transportation systems offers safer traveling, reduced vehicle collisions, increased access to public transportation, improved traffic management, and support for infotainment. Having a network of connected autonomous vehicles with multiple sensors requires 6G to provide huge levels of reliability (99.99999% uptime) and low latency (below 1 ms) [8]. Adding more edge-computing servers and locations will help enable this capability to ensure passenger safety and provide the ability to relay real-time information to other vehicles. In case of an accident, timely information can be provided to the police and first responders automatically, and other vehicles can be directed away from the accident, minimizing injuries and the amount of traffic.
UASs and Unmanned Aerial Vehicles (UAVs)
An important aspect of wireless networks beyond 5G and 6G in the next decade will be the widespread and increasing usage of UASs. Wireless communication must be dependable, secure, and cost-effective to enable their vast deployment [3]. To facilitate highly reliable connections and maintain availability 99.99999% of the time, UASs and UAVs will have to implement 3-D wireless connectivity and the use of terrestrial and nonterrestrial networks. Depending on location, altitude, and presence of line of sight, UAVs will be able to maintain communication with a 6G base station or a satellite. On top of being able to choose which connection type, UASs deployed together can act as nodes within their own “mesh” network. This means UASs will be able to communicate between each other to relay data or pick up signals as long as a node in the systems can maintain connection to a network. Implementing AI/ML and edge-computing features into UASs also leads us to a more connected future. AI at the edge allows for the supervision and control of inter-UAS communication systems for all terminals and creates an intelligent cloud that can facilitate the coordination of big data to make decisions on the fly (Figure 5). For example, a UAV’s image processing unit can be used to conduct surveillance, reconnaissance, or inspections; alert a central command station autonomously; and follow a target until an operator arrives. Having a quicker, more intelligent, and reliable network will be pivotal to future military operations. UASs will provide the tactical capabilities for medical evacuation, cargo and resupply, intelligence, surveillance, aerial refueling, air-to-air combat, strategic bombing, battle management and command and control, electronic warfare, swarming, and the suppression and destruction of enemy air defense with lethal autonomous weapons. UASs will also be useful in case of an accident, collision, crime, or other incident to send the appropriate personnel as soon as possible.
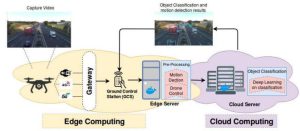
Figure 5. Overview of the Computation Offloading Scheme on Drone-Based Video Analytics (Source: https://par.nsf.gov/servlets/purl/10131891).
2.3.2 Smart Healthcare System and Biomedical Communications
The world’s medical health systems will also benefit from the expected advancements of 6G. 6G’s ability to maintain a high-data rate, low latency, and uptime of 99.99999%, paired with the implementation of edge computing and AI/ML, will help reduce time and space barriers associated with receiving access to care. Modern health care applications that expect to utilize 6G’s improvements include emergency services, insurance services, ambulance services, hospital-to-home services, pharmacy services, remote surgeries from doctors, and intelligent wearable devices (IWDs). Using IWDs will help patients quickly and efficiently conduct continuous monitoring of their health for clinical diagnostics and analysis, athletics, and human-machine interfaces. Examples of information transmitted by these in-body sensors include heartbeat rate, health conditions like diabetes, blood test and pressure, body weight, and nutrition. Coupled with AI capabilities, these sensors can be used to send updates and alerts to the patient or health professionals to keep them informed on health status remotely. The in-body sensors with batteryless communication technologies are desirable for reliable and long-term monitoring [9]. Due to the tremendously sensitive quality of service requirements within the health industry, today’s network capabilities are unable to facilitate services like remote robotic surgeries. 6G’s increased reliability, ultra-low latency, and mobility support make conducting remote robotic surgeries a possibility in the future, while ensuring patient safety. A smart health care system will help make patients’ lives more manageable by lowering the amount of hospital visits and keeping them informed on their health during an emergency or on a regular day.
2.3.3 Extended Reality
Extended reality (XR) services represent virtual reality (VR), augmented reality (AR), and mixed reality (MR). VR is a computer-simulated, 3-D experience in which computer technologies use reality headsets to generate realistic sensations and replicate a real environment or create an imaginary world. An actual VR environment engages all five senses.
AR is a live view of a real physical world whose elements are augmented by various computer-generated sensor inputs, such as audio, video, visuals, and global positioning system data. It uses the existing reality and adds to it by using an AR headset or glasses and software.
Sometimes called a hybrid reality, MR merges the real and virtual worlds to create new atmospheres and visualizations to interact in real-time. One critical characteristic of MR is that artificial and real-world content can respond to one another in real-time.
XR refers to all combined real and virtual environments and human-machine interactions generated by computer technology and wearables [9].
The increased use of streaming services and multimedia applications has justified adopting and implementing millimeter-wave technology and 5G. This ability to support more data at once has attracted new applications that are even more data heavy than the average multimedia content and triggered the early adoption of AR and VR. Eventually, these immersive experiences and services will deplete 5G networks and require a network like 6G that can provide a capacity of 1 TB/s or higher to meet the latency and reliability requirements that enable true real-time user interaction.
XR services will have applications in many domains such as telemedicine, haptics, flying vehicles, brain-computer interfaces, connected autonomous systems, and entertainment like gaming, multimedia, and sports. Overall, XR will provide vastly more immersive experiences for the user that can help bridge the gap between communicating with people or operating systems from anywhere.
2.3.4 Industry 4.0 and Robotics
The industry 4.0 revolution started with 5G, which is the digital transformation of cyber-physical, system-based manufacturing processes using automation, data exchange, and IoT services. 6G will help overcome the boundaries between the real factory and the cyber computational space, enabling internet-based diagnostics, maintenance, operation, and direct machine communications in a cost-effective, flexible, and efficient way. Industrial and critical infrastructure controls require real-time operations with guaranteed uptime of 99.99999% and gigabit-per-second peak data rates.
These factories can enhance the manufacturing process (Figure 6), balance between the market’s supplies and demand, and predict a machine’s maintenance needs. Substantial global companies can monitor their factories remotely through global control centers. The companies can also predict the failure and success of a product line flow based on historically collected data [3]. For example, a critical industrial system may need to regulate a faulty IoT device with no delay or operators may need to control robotics in a factory at real-time to complete a task. The improved features of 6G will accelerate the advancement of industry 4.0, leading to a smarter supply chain, quality control improvements, efficient environmental monitoring, and automation using AI-based, machine-to-machine communication, energy harvesting, and backscatter communications.
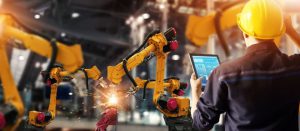
Figure 6. An Advanced Robot for Manufacturing (Source: https://www.dodmantech.mil/Manufacturing-Innovation-Institutes/ARM-Institute/).
2.3.5 Holographic Telepresence
The human tendency and desire to connect remotely with increasing fidelity will pose severe communication challenges in 6G networks. Using a hologram or 3-D holographic display in their everyday lives has been the dream of many. The data rate requirements of a 3-D holographic display—a raw hologram without any compression and with colors, full parallax, and 30 fps—would require 4.32 TB/s [11]. The latency requirements of a 3-D holographic display would also be below 1 ms. Thousands of synchronized view angles will be necessary, as opposed to the few required for VR/AR. 6G’s ability to provide data rates over 1 TB/s, low latency, and improved reliability may allow for this new media format to emerge. Moreover, to fully realize an immersive remote experience, all five human senses are destined to be digitized and transferred across future networks, increasing the overall target data rate [8].
References
[1] BBC. “China Sends ‘World’s First 6G’ Test Satellite into Orbit.” https://www.bbc.com/news/av/world-asia-china-54852131, 7 November 2020.
[2] U.S. DHS. “5G/6G Wireless Networks.” https://www.dhs.gov/science-and-technology/5g6g, 2 February 2023.
[3] Abdel Hakeem, S. A., H. H. Hussein, and H. Kim. “Vison and Research Directions of 6G Technologies and Applications.” Journal of King Saud University – Computer and Information Sciences, vol. 34, no. 9, pp. 2419–2442, https://www.sciencedirect.com/science/article/ pii/S1319157822001033, 20 June 2022.
[4] Weedon, A. “Huawei Is Beginning 6G Research – a Mobile Network That May Move Far Beyond Smartphones.” https://www.abc.net.au/news/2019-08-22/huawei-reportedly-entering-into-6g-research/11427056, 21 August 2019.
[5] IHS Markit. “Number of Connected IoT Devices Will Surge to 125 Billion by 2030, IHS Markit Says.” https://news.ihsmarkit.com/prviewer/release_only/slug/number-connected-iot-devices-will-surge-125-billion-2030-ihs-markit-says, 24 October 2017.
[6] Qadir, Z., K. N. Le, N. Saeed, and H. Suliman Munawar. “Towards 6G Internet of Things: Recent Advances, Use Cases, and Open Challenges.” https://www.sciencedirect.com/ science/article/pii/S2405959522000959#b83, 20 June 2022.
[7] Rahman, I., S. Moddarres Razavi, O. Liberg, C. Hoymann, H. Wiemann, C. Tidestav, P. Schliwa-Bertling, P. Persson, and D. Gerstenberger. “5G Evolution Toward 5G Advanced: An Overview of 3GPP Releases 17 and 18.” https://www.ericsson.com/en/reports-and-papers/ericsson-technology-review/articles/5g-evolution-toward-5g-advanced, 13 October 2021.
[8] Giordani, M., M. Polese, M. Mezzavilla, S. Rangan, and M. Zorzi. “Toward 6G Networks: Use Cases and Technologies” IEEE Communications Magazine, vol. 58, no. 3, pp. 55–61, https://ieeexplore.ieee.org/abstract/document/9040264, 18 March 2020.
[9] Chowdhury, M. Z., M. Shahjalal, S. Ahmed, and Y. M. Jang. “6G Wireless Communication Systems: Applications, Requirements, Technologies, Challenges, and Research Directions.” IEEE Open Journal of the Communications Society, vol. 1, pp. 957–975, https://ieeexplore. ieee.org/abstract/document/9144301, 20 July 2020.
[10] Shepardson, D., and B. Klayman. “U.S. Government to End Gas-Powered Vehicle Purchases By 2035 Under Biden Order.” https://www.reuters.com/world/us/biden-pledges-end-gas-powered-federal-vehicle-purchases-by-2035-2021-12-08/, 3 December 2021.
[11] Xu, X., Y. Pan, P. Y. Lwin, and X. Liang. “3D Holographic Display and Its Data Transmission Requirement.” https://ieeexplore.ieee.org/document/6122872, 23 October 2011.
Want to find out more about this topic?
Request a FREE Technical Inquiry!